Researchers say this combo of old-school invisible ink and AI is ‘uncrackable’
A team of researchers from the Harbin Institute of Technology in China have developed a system by which invisible ink and a basic cipher can be combined with relatively simple AI to create an ‘uncrackable’ offline encryption method.
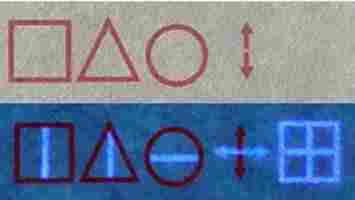
Up front: Remember when you learned that you could use lemon juice and water to write invisible messages on paper? Well, this is a lot like that. The researchers then take the invisible ink and use it to write a code that only an AI can break.
Per the team’s paper :
Background: Technology holds the promise of ‘unbreakable’ encryption in the form of quantum communications or some other non-reproducible form of data carriage. But this is not that.
This research showcases how basic technologies can be combined to produce something greater than the sum of their parts. Artificial intelligence is a backbone technology for modern encryption, but it’s traditionally unhelpful if you’re trying to keep prying eyes out of your physical documents (such as paper).
It’s unclear why you’d have a cache of secret documents that can’t be encrypted in digital form… perhaps you’re a spy who has to pass handwritten notes to avoid Big Brother and the Eye In The Sky .
Nevertheless, plain invisible ink messages can be intercepted and exposed without the use of technological aids and visible codes are easily spotted as aberrant.
With this paradigm, you could come into physical possession of the document without realizing it had invisible ink – the team’s process results in ink that’s virtually impossible to detect without the use of a UV light. And, even if you discovered the ink, it wouldn’t do you any good because the message would also be written in a code that could only be broken with the code book.
Quick take: Technically speaking, there’s no reason why AI has to be involved in this encryption system. Any two humans could share the code book and use the chemical-based invisible ink process to send messages back and forth. But if you try to scale such an operation, it eventually becomes unwieldy. You can only have so many copies of the code book floating around before the encryption model is no longer secure. People suck at keeping secrets.
But AI won’t lose the book or sell it to the enemy or a competitor. Given all the scary use cases for something like this, that seems important.
Read the whole paper here .
100 years of quantum physics: From 1920s theories to mind-bending computers of 2020
Back in the 1920s, quantum mechanics, which is the theory that underpins everything from how atoms behave to how quantum computers work, was well on the way to gaining mainstream acceptance. But one conundrum remained: Sometimes, quantum objects like electrons, atoms, and molecules behave like particles, and others they behave like waves. Sometimes they even behave like particles and waves at the same time. So, when studying these quantum objects, it has never been quite clear which approach scientists needed to use for their calculations.
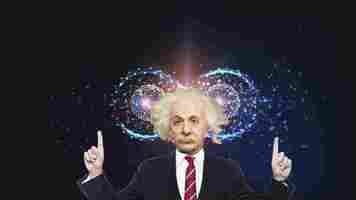
Sometimes, scientists needed to assume that the quantum objects were waves to get the correct result. Other times, they needed to assume that the objects were, in fact, particles. Sometimes either approach would work. But other times only one approach would lead to the right result, and the other approach returned a bogus one. The history of this problem goes back quite a bit, but recent experiments have shed some new light on this old question.
Quantum history
In the eponymous double-slit experiment, first performed by Thomas Young in 1801, light behaved like waves. In this experiment, one points a laser beam at a double slit and then looks at the pattern that results from it. If light were made of particles, one would expect two blocks of light, in the shape of the slits. Instead, the result is lots of little blocks of light, arranged in a characteristic order. Placing a double slit in a stream of water would result in the same pattern a little further down. Hence, this experiment led to the conclusion that light is a wave.
Then, in 1881, Heinrich Hertz made a funny discovery . When he took two electrodes and applied a high enough voltage between them, sparks resulted. So far, so good. But when Hertz shone a light on those electrodes, the sparking voltage changed. The explanation was that the light knocked electrons out of the electrode material. But, curiously enough, the maximum speed of the knocked-out electrons didn’t change if the intensity of the light varied, but instead changed with the frequency of the light. This result should have been impossible if wave theory was true. In 1905, Albert Einstein had the solution: Light was, in fact, a particle.
All of this was unsatisfactory. Scientists prefer one theory that is always right to two theories that are sometimes right. And if a theory is only sometimes right, then we would like, at the very least, to be able to tell under which conditions it is right.
But that was exactly the problem with this discovery. Physicists didn’t know when to treat light, or any other object, as a wave or when as a particle. They knew that some things trigger wave-like behavior, such as the edges of slits. But they didn’t have a clear explanation why that is the case, or when to use which theory.
This conundrum, called wave-particle duality, persists to this day. But new research might be clearing things up a bit. Scientists at Korea’s Institute for Basic Sciences have shown that the properties of the light source influence how much it is a particle and how much it is a wave. Through a novel approach to exploring this problem, they’ve paved a way that might even lead to improvements in quantum computing. Or so are the hopes.
How to make particles and waves
In the experiment, scientists used a semi-reflective mirror to split a laser beam into two parts. Each of these beams hits a crystal, which, in turn, produces two photons. This produces four photons in total, two from each crystal.
The scientists sent one photon from each crystal into an interferometer. This device merges two sources of light and creates an interference pattern. Such a pattern was first observed by Thomas Young in his aforementioned double-slit experiment. It’s also what you see when you drop two stones in a pond: overlapping ripples of water, some of which heighten each other and others that cancel each other out. In other words, the interferometer detects the wave nature of light.
The paths of the other two photons were used to detect their particle characters. Though the authors of the paper don’t specify how they did that, this is generally done by shooting the photon through a material that marks where the photon went. For example, one can shoot the photon through gas, which then lights up where the photon went. By focusing on the trajectory rather than the end destination, the photon can’t be a wave. This is because if one measures the exact location of the photon at every instant in time, it’s pointlike and can’t interfere with itself.
This is one of the many examples in quantum physics where the measurement actively influences the outcome of said measurement. Hence, in this part of the experiment, there was no interference pattern at the end of the photon’s trajectory. The researchers thus revealed how a photon could be a particle. The challenge was now to quantify how much it was a particle, and how much of the wave character remained.
Because both photons of one crystal are produced together, they form a single quantum state. This means that one can find a mathematical formula that describes both of these photons at the same time. As a result, if the researchers can quantify how strong the “particleness” and the “waviness” of the two photons are, then this quantification applies to the whole beam that arrives at the crystal.
Indeed, the researchers managed just that. They measured how wavy the photon was by checking the visibility of the interference pattern. When the visibility was high, the photon was very wavelike. When the pattern was barely visible, they concluded that the photon must be very particle-like.
And this visibility wasn’t random. It was highest when both crystals got an equal intensity of the laser beam. If, though, one crystal’s beam was much more intense than the other, the visibility of the pattern got very faint and the photons were most likely particle-like.
This result is exciting because most experiments only measure light as either waves or as particles. Few experiments to date have measured both at the same time. Doing so means it’s easier to determine how much of each property a light source has.
Theoretical physicists are excited
This result matches the prediction that theorists made earlier. According to their theory, how wavelike and how particle-like a quantum object is depends on the purity of the source. Purity, in this context, is just a fancy way of expressing the likelihood that a particular crystal source will be the one that emits light. The formula goes as follows: V2 + P2 = µ2, where V is the pattern visibility, P is the path distinguishability, and µ is the source purity.
This means that a quantum object, such as light, can be to some extent wavelike and to some extent particle-like, but this is limited by how pure the source is. A quantum object is wavelike if an interference pattern is visible or if the quantity V is not zero. Also, it is particle-like if a path is distinguishable or if P is not zero.
Another consequence of this prediction is that the purity is that if the quantum-path entanglement is high, the purity is low, and vice versa. The scientists who conducted the experiment showed this mathematically in their paper. By tuning the crystals’ purity and measuring the results, they were able to show that these theoretical predictions are indeed valid.
Faster quantum computers?
The connection between a quantum object’s entanglement and its particleness and waviness is particularly exciting. Quantum devices, which might one day power the quantum internet , rely on entanglement. The quantum internet is the quantum analogy to what the internet is for classical computers. By stringing lots of quantum computers together and letting them communicate, scientists hope to unlock more power than a single quantum computer could ever achieve.
But instead of sending bits through optical fiber, which is what we do to power the classical internet, we need to entangle qubits to form a quantum internet. Being able to measure entanglement by a photon’s particleness and waviness means that we may find easier ways to control the quality of the quantum internet.
In addition, quantum computers themselves might get better using particle-wave duality. According to a proposal by researchers with China’s Tsinghua University, one could shoot a small quantum computer through a multi-slit to enhance its power. A small quantum computer would consist of several atoms, which themselves are used as qubits, and such devices already exist .
Shooting these atoms through a multi-slit is a lot like shooting light through a double-slit, albeit slightly more complex, of course. This would create more possible quantum states, which in turn enhances the power of the “shot” computer. The mathematics behind all of this is too much to explain in this article, but an important result is that such a dual-quantum computer might be better in parallel computing than regular quantum computers. Parallel computing is common in classical computing, too, and basically refers to the capacity of a computer to carry out several calculations at the same time, thus making it faster overall.
So, although this is very fundamental research, possible applications are already on the horizon. It’s impossible to prove at this point in time, but these findings might speed up quantum computers even further and make the quantum internet happen a little sooner.
Very fundamental, but very exciting
All of this should be taken with a big grain of salt. The research is solid, but it’s also very fundamental. As usual in science and technology, it’s a long way from fundamental research to real-life applications.
But the researchers from Korea did find one very interesting thing: The conundrum about wave-particle duality isn’t going away any time soon. Quite on the contrary, it seems to be so deeply embedded in all quantum objects that it might be better to make use of it. With a new quantitative footing, linked to the purity of the source, this will be easier to do.
One of the first use cases might happen in quantum computing. Quantum entanglement and wave-particle duality are linked, as scientists have shown. Instead of entanglement, one could therefore measure the quantities of waviness and particleness. This could help the scientists who are working on developing the quantum internet. Or, one could use the duality to enhance quantum computers and make them even faster. Either way, it seems that exciting quantum times are soon to be upon us.
This article was originally published on Built In. You can read it here .
Oh no… Mark Zuckerberg wants to build a metaverse
Imagine living in a virtual space that merges the physical and digital worlds — and is built by Facebook.
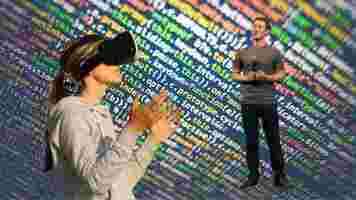
It may sound like the stuff of nightmares, but Mark Zuckerberg hopes to make it a reality. Yup, the 37-year-old billionaire wants to build a metaverse.
“Yo u can think about the metaverse as an embodied internet, where instead of just viewing content — you are in it,” he told The Verge .
In Zuckerberg’s vision, the metaverse will be accessible across VR, AR, PCs, mobile devices, and game consoles:
Inside the metaverse, Zuckerberg imagines us jumping into 3D concerts from our phones and sitting together as holograms on couches.
“This is something that I’m spending a lot of time on, thinking a lot about, we’re working on a ton,” he said.
The concept of a metaverse is intriguing… but I don’t want Facebook to build it.
Life in the metaverse
An immersive metaverse could supercharge the misinformation, surveillance, and harassment that’s already rampant on social networks.
Facebook’s data harvesting may also become vastly more intrusive, as VR expert Verity McIntosh told the BBC :
Facebook’s track record doesn’t make me optimistic that our privacy will be protected. I’m also wary of how ads will be delivered in the metaverse, and its potential to exacerbate the digital divides that deny many people access to services.
[Read next: Opinion: Facebook’s brain computer interface will be the instrument of society’s collapse ]
Zuckerberg does say he wants the metaverse to be an interoperable and open system that’s run by multiple companies and creators. But Facebook’s history of buying rivals and stifling competition makes me skeptical about his claims.
Even if the virtual space is operated collaboratively, I’d be concerned about how it’s governed. Tech firms aren’t generally fans of external regulation.
None of this is to say that a metaverse is a bad idea. After 18 months of pandemic life, the idea of teleporting to a virtual island or catching up with holograms of family abroad is incredibly attractive. I just hope that Facebook won’t be watching our every move.
Greetings Humanoids! Did you know we have a newsletter all about AI? You can subscribe to it right here .